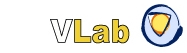 |
News |
|
We are delighted to share that on May 10, 2024, we had a wonderful dinner together to
celebrate Qi Zhang’s graduation.
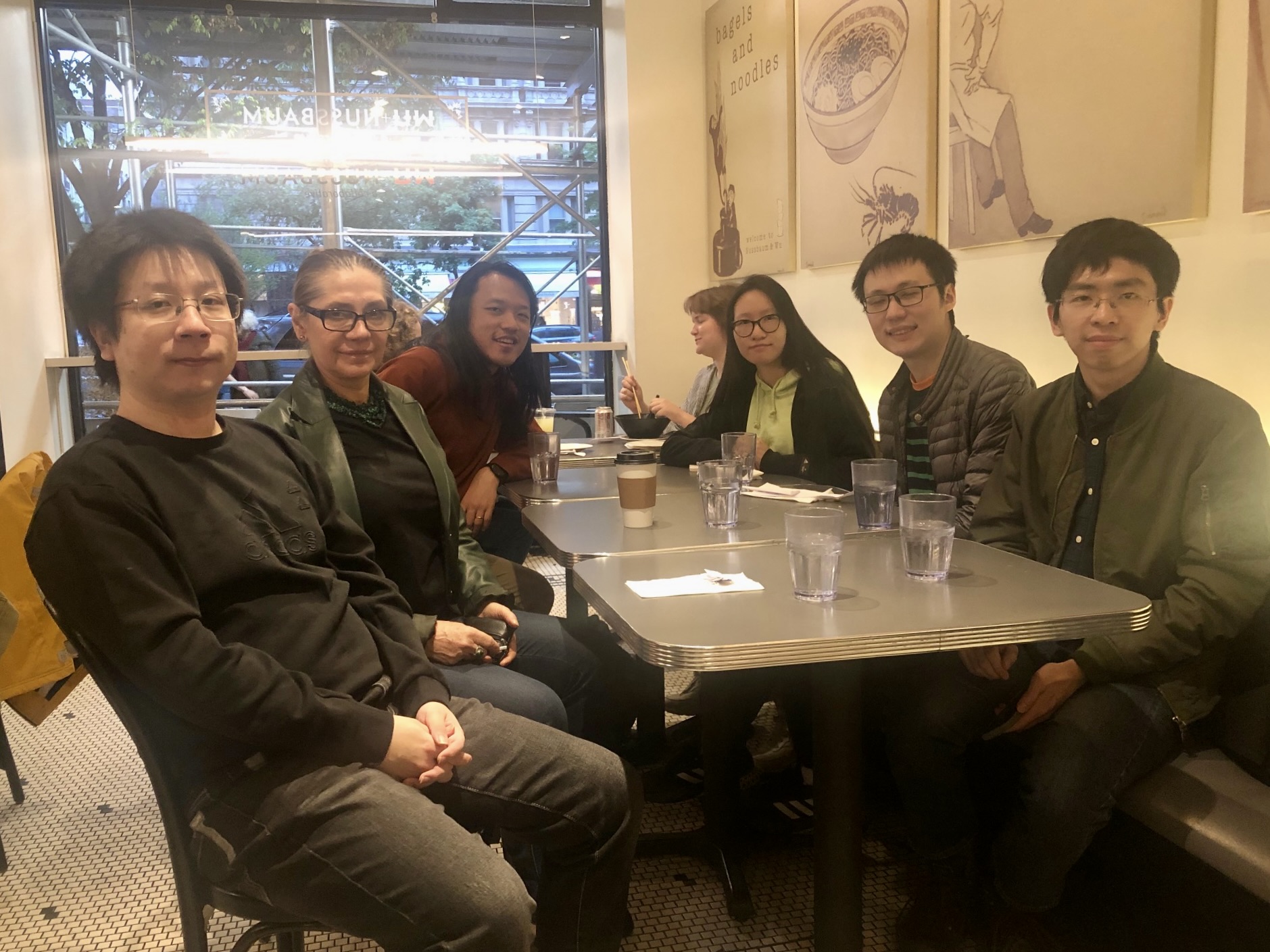
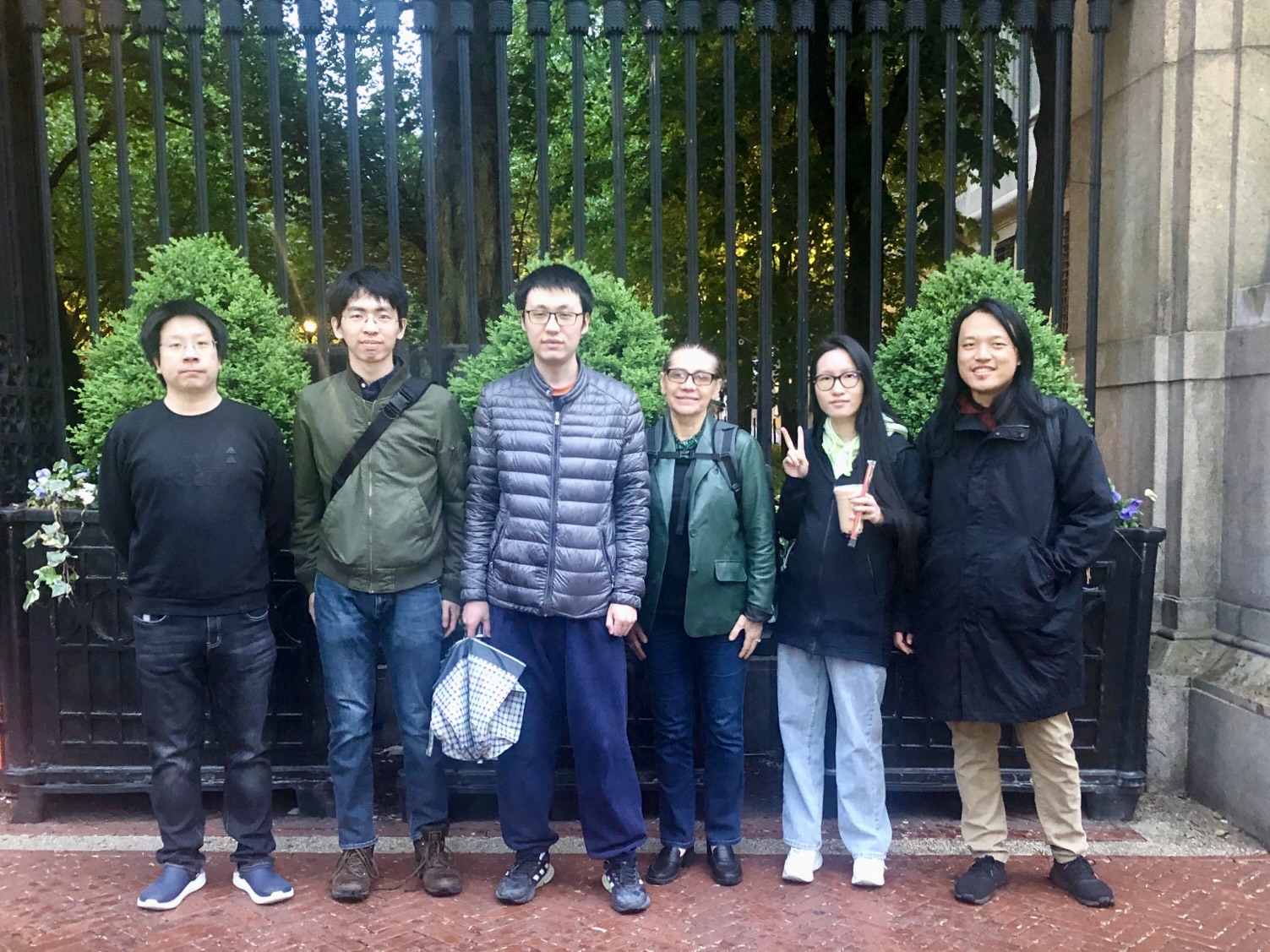
Congratulations, Qi!
We are proud to announce that Qi Zhang has successfully defended his thesis and has
earned his Ph.D.
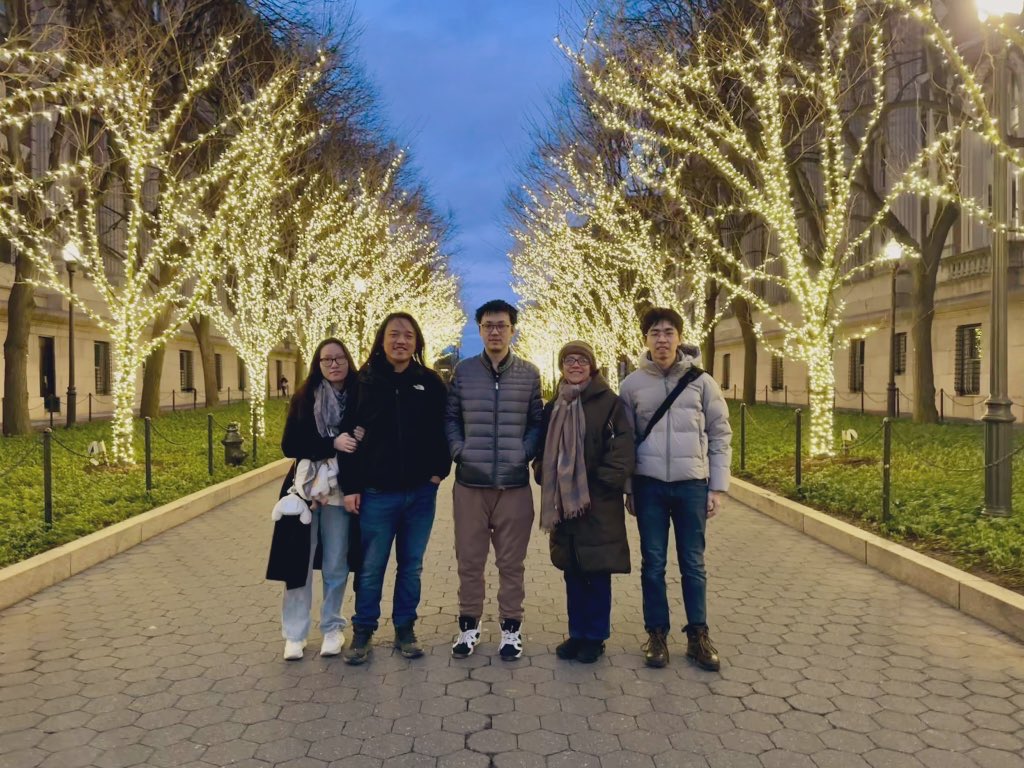
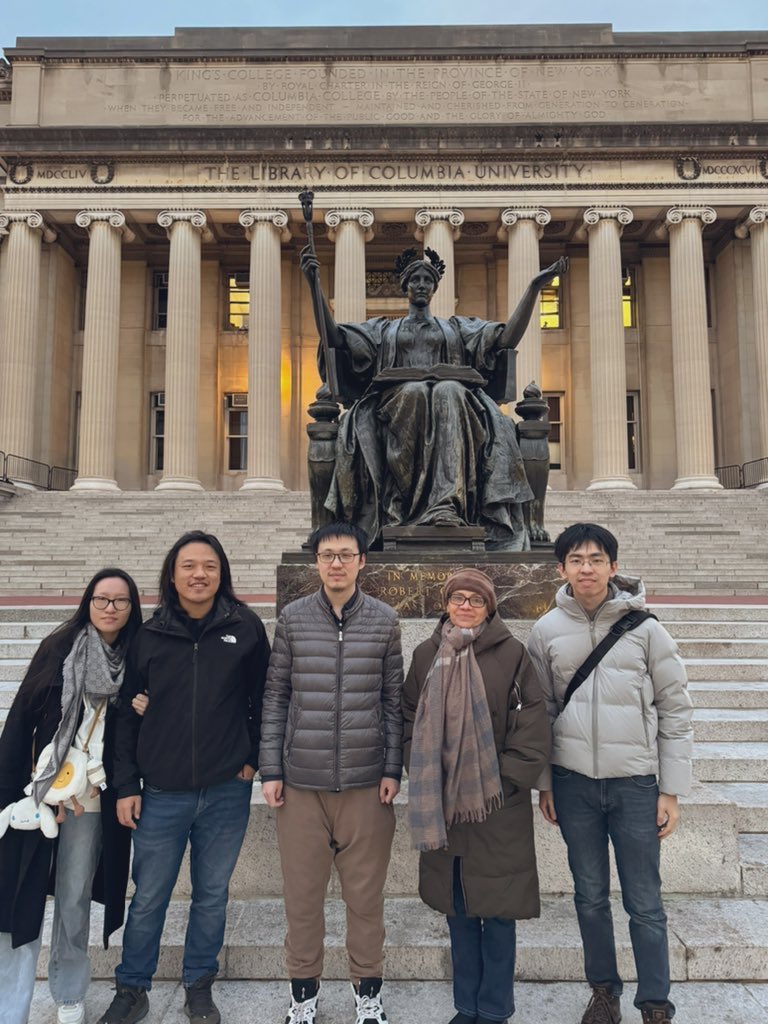
Hard work pays off, and you’ve proved it. Super proud to have you in our crew. Here’s
to your bright future!
Join us in our Twitter feed for celebration!
With a heavy heart, we communicate the passing of Prof. David. A. Yuen. His upbeat
optimism, creativity, geophysical insights, and friendship will be deeply missed. A
great loss to geophysics.
Dave, you were one of a kind!
We are proud to announce that Zhen Zhang has successfully defended his thesis and has
earned his Ph.D.
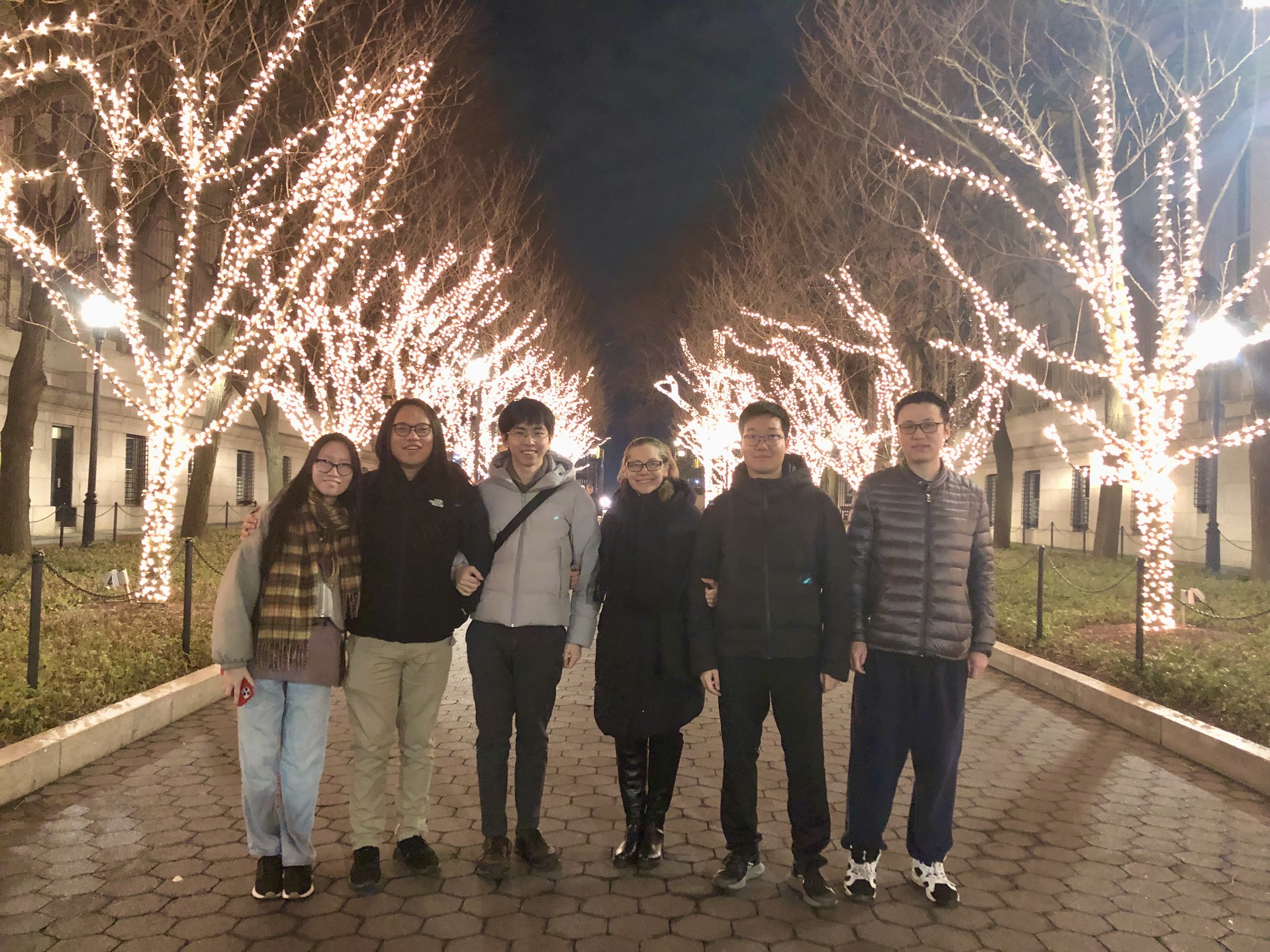
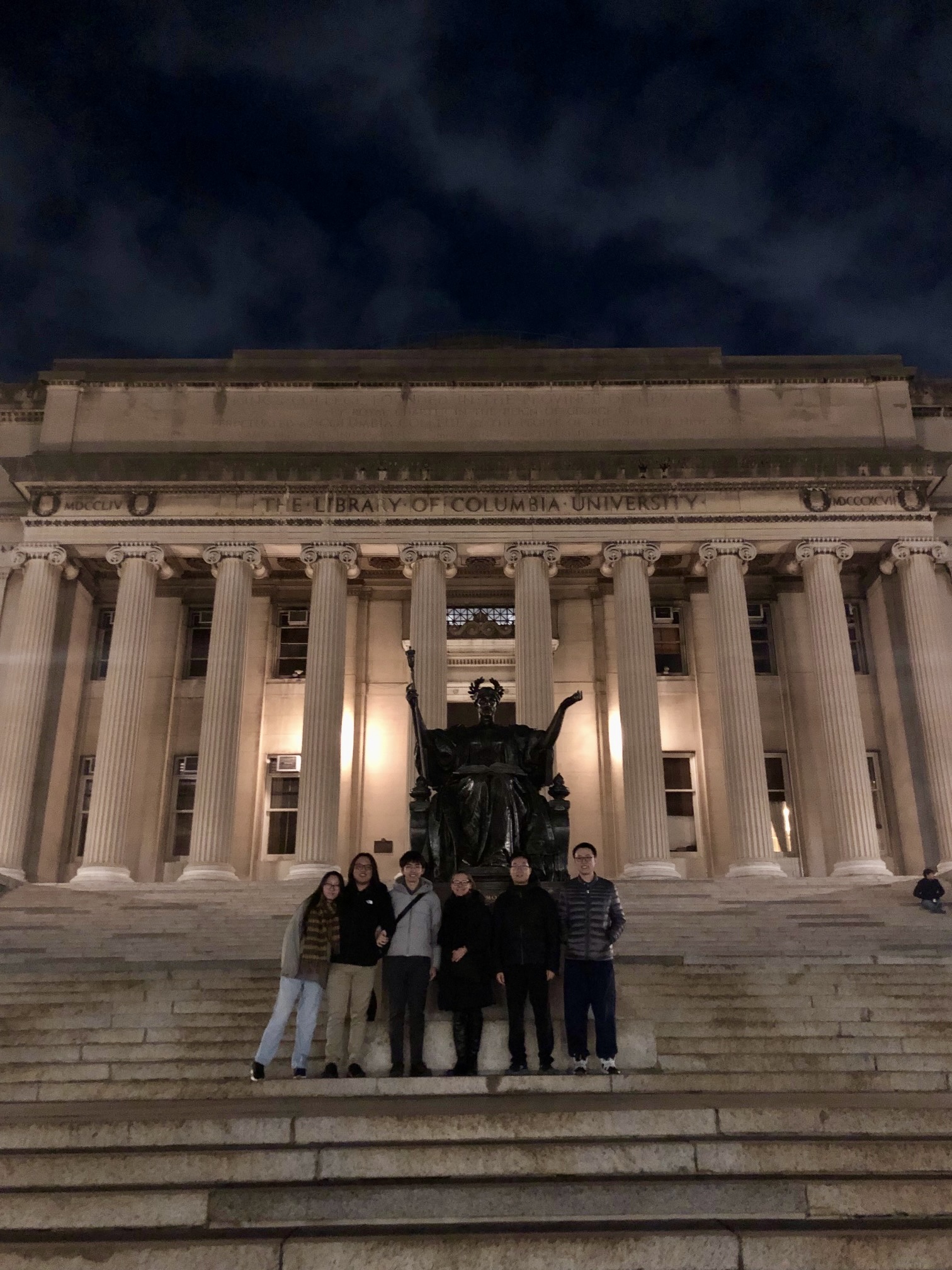
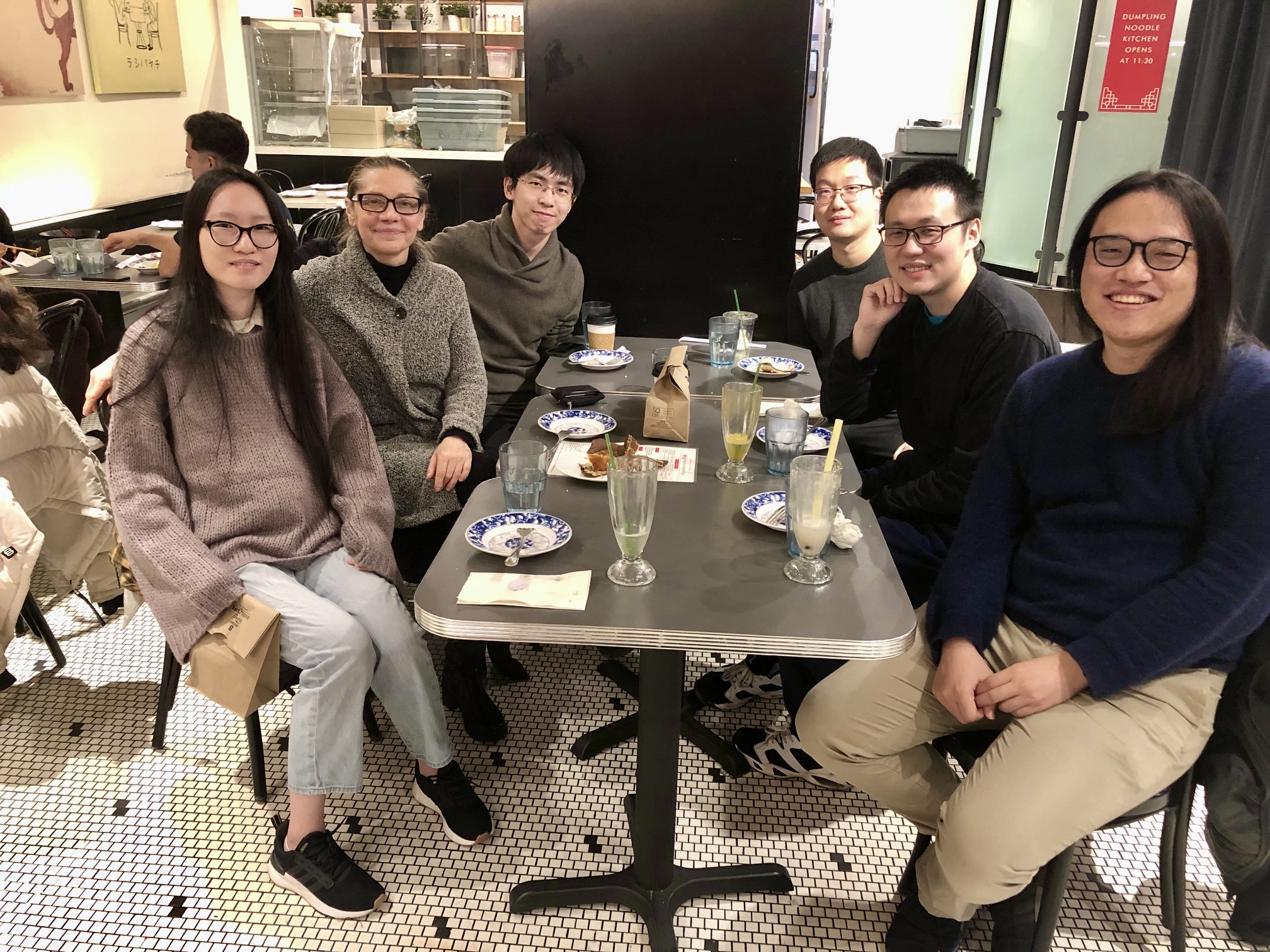
We wish him all the best in his future endeavors and look forward to seeing the
impact of his research in the field.
Congratulations once again, Zhen Zhang!
Join us in our Twitter feed for celebration!
Renata Wentzcovitch, professor of material science, applied physics, and earth and
environmental sciences, has been named president-elect for the American Geophysical
Union. (Read More)
Press Highlights
What is it about?
FeO is a compound of great interest in condensed matter physics and geophysics. It
has complex and subtle structural, magnetic, and electronic transitions. It has been
challenging for theoretical/computational methods to address such property changes
in a prototypical, strongly correlated material such as FeO. This paper shows that
the fundamental properties of FeO can be described successfully at high pressures
and temperatures by a standard density-functional-based method once its dynamic
complexity and electronic excitations are addressed simultaneously.
Why is it important?
This work establishes the theoretical framework to predict the properties of iron
alloys at the extreme thermodynamic conditions of the Earth’s core, an enigmatic
planet region. This framework should be a starting point for investigating the
properties of other alleged strongly correlated materials at more normal
thermodynamic conditions.
Perspectives
Several theoretical/computational methods needed to be developed to address
diverse challenges before a full-scale simulation of this complex material could
be performed successfully under such extreme pressure and temperature
conditions. The authors used a novel combination of approaches and methods
developed in-house to perform these simulations.
– Renata Wentzcovitch
A quantum phase transition called spin crossover can be used to visualize
deep-Earth processes like subducting tectonic plates. Photo credit: Nature
Communications
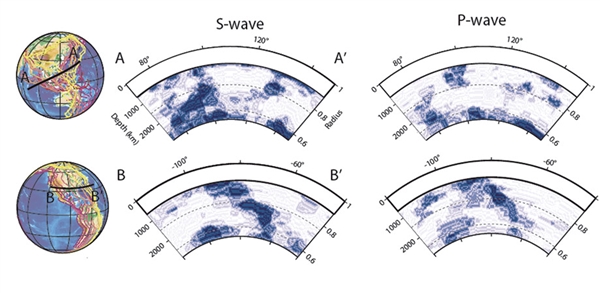
Shephard, G.E., Houser, C.,et al., Wentzcovitch, R.M.,
Seismological expression of the iron spin crossover in ferropericlase in the
Earth’s lower mantle. Nat Commun 12, 5905 (2021). https://doi.org/10.1038/s41467-021-26115-z
Click to preview the magazine PDF.
A new study investigates iron’s form at the planet’s interior. The findings have
repercussions for understanding the inner core’s structure.

Y. Sun, M. I. Mendelev, F. Zhang, Z. Liu, B. Da, C.-Z Wang,
R. M. Wentzcovitch, and K.-M. Ho. Geophys. Res. Lett. (2023).
https://doi.org/10.1029/2022GL102447
Press Highlights
Earth’s inner core is dominated by iron, which can exist as a solid material in
more than one crystallographic form. (This quality allows iron to combine with
other elements to form alloys.) Iron’s most stable form at room temperature is
the body-centered cubic (bcc) structure. At extremely high pressures, it is
stable in its hexagonal close-packed (hcp) phase. Of considerable debate,
however, is iron’s structure at the center of Earth. In a new study, Sun et al.
get one step closer to an answer.
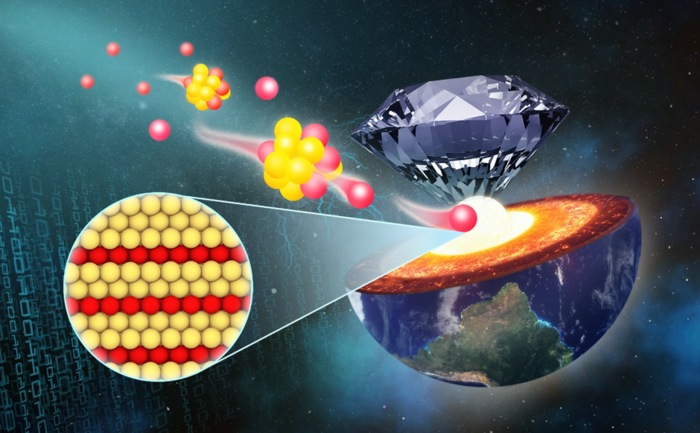
J. Liu, Y. Sun, C. Lv, F. Zhang, S. Fu, V. B. Prakapenka, C.-Z.
Wang, K.-M. Ho, J.-F. Lin, R. M. Wentzcovitch. The Innovation
(2022). https://doi.org/10.1016/j.xinn.2022.100354
Press Highlights
Oxygen is the key substance for life and one of the most abundant elements in the
Earth. However, it’s still unknown whether oxygen is present and in which form
in the inner core with extreme high pressure and temperature conditions, and
almost composed of pure iron. Scientists co-led by Dr. Jin Liu from HPSTAR (the
Center for High Pressure Science &Technology Advanced Research) and Dr. Yang
Sun from Columbia University reveal that Fe-rich Fe-O alloys are stable at
extreme pressures of nearly 300 GPa and high temperatures of more than 3,000 K.
The results published in the journal of The Innovation prove that oxygen can
exist in the solid inner core, which provides key constraints for further
understanding of the formation process and evolution history of the Earth’s
core.
Is the Earth’s inner core so “anoxic?” To answer this question, a series of
experiments and theoretical calculations were carried out in this study.
To be close to the temperature and pressure of Earth’s core, pure iron and iron
oxide were placed on the tips of two diamond anvils and heated with a
high-energy laser beam. After many attempts, it was found that a chemical
reaction between iron and iron oxide occurs above 220–260 GPa and 3,000 K. The
XRD results reveal that the reaction product is different from the common
high-temperature and high-pressure structure of pure iron and iron oxide.
This interdisciplinary study confirms predictions made by Wentzcovitch and group
members in 2014 that lateral temperature variations in the lower mantle produce an
anti-correlation between bulk and shear velocities owing to the spin crossover in
iron in ferropericlase. This effect provides an interpretation for previously
mysterious observations in seismic tomography maps.
Shephard, G.E., Houser, C.,et al., Wentzcovitch, R.M.,
Seismological expression of the iron spin crossover in ferropericlase in the
Earth’s lower mantle. Nat Commun 12, 5905 (2021). https://doi.org/10.1038/s41467-021-26115-z
Press Highlights
Researchers have identified a quantum phase transition taking place in iron more
than 1000 kilometres deep within the Earth’s mantle. This transition, known as a
spin crossover, also occurs in nanomaterials used for recording information
magnetically, meaning that the effect stretches from the macro- to the
nanoscale.
Cold, subducting oceanic plates are seen as fast velocity regions in (a) and (b),
and warm rising mantle rock is seen as slow velocity regions in (c). Plates and
plumes produce a coherent tomographic signal in S-wave models, but the signal
partially disappears in P-wave models. (Courtesy: Columbia Engineering)
In 2006, Columbia Engineering Professor Renata Wentzcovitch published her first
paper on ferropericlase, providing a theory for the spin crossover in this
mineral. Her theory suggested it happened across a thousand kilometers in the
lower mantle. Since then, Wentzcovitch, who is a professor in the applied
physics and applied mathematics department, earth and environmental sciences,
and Lamont-Doherty Earth Observatory at Columbia University, has published 13
papers with her group on this topic, investigating velocities in every possible
situation of the spin crossover in ferropericlase and bridgmanite, and
predicting properties of these minerals throughout this crossover. In 2014,
Wenzcovitch, whose research focuses on computational quantum mechanical studies
of materials at extreme conditions, in particular planetary materials predicted
how this spin change phenomenon could be detected in seismic tomographic images,
but seismologists still could not see it.
Click to extend...
Working with a multidisciplinary team from Columbia Engineering, the University
of Oslo, the Tokyo Institute of Technology, and Intel Co., Wenzcovitch’s latest
paper details how they have now identified the ferropericlase spin crossover
signal, a quantum phase transition deep within the Earth’s lower mantle. This
was achieved by looking at specific regions in the Earth’s mantle where
ferropericlase is expected to be abundant. The study was published October 8,
2021, in Nature Communications.
“This exciting finding, which confirms my earlier predictions, illustrates the
importance of materials physicists and geophysicists working together to learn
more about what’s going on deep within the Earth,” said Wentzcovitch.
Spin transition is commonly used in materials like those used for magnetic
recording. If you stretch or compress just a few nanometer-thick layers of a
magnetic material, you can change the layer's magnetic properties and improve
the medium recording properties. Wentzcovitch’s new study shows that the same
phenomenon happens across thousands of kilometers in the Earth’s interior,
taking this from the nano- to the macro-scale.
“Moreover, geodynamic simulations have shown that the spin crossover invigorates
convection in the Earth’s mantle and tectonic plate motion. So we think that
this quantum phenomenon also increases the frequency of tectonic events such as
earthquakes and volcanic eruptions,” Wentzcovitch notes.
Our planet is full of mysteries. How exactly did Earth form and evolve to its
current state? Why do some places in its interior seem hotter or colder, rising
or sinking? For answers, geoscientists experiment on materials expected to be
found in Earth’s interior, but these exist at immense pressures and temperatures
that are impractical to reproduce in the lab. Renata Wentzcovitch, a condensed
matter physicist, says quantum simulations can help.
“Nature is quantum,” said Wentzcovitch, a professor at Columbia Engineering and
the Lamont Doherty Earth Observatory.
Science Highlights
Ferropericlase, one of the primary mantle minerals, is known to have an
electronic spin transition. However, evidence of this transition in the Earth’s
mantle has been challenging to find. Shephard et al. found changes in seismic
wave speeds at two depth ranges that correspond to the iron spin transition in
ferropericlase. The authors compared compressional and sheer-wave velocities in
tomographic models, finding relative changes between the two types of waves that
they could attribute to the transition. Using tomographic models is important
because nonuniform thermochemical variations wash the signal out in global,
one-dimensional models.
Nat. Commun. 12, 5905 (2021).
Follow us in Twitter for more research updates!
|
|